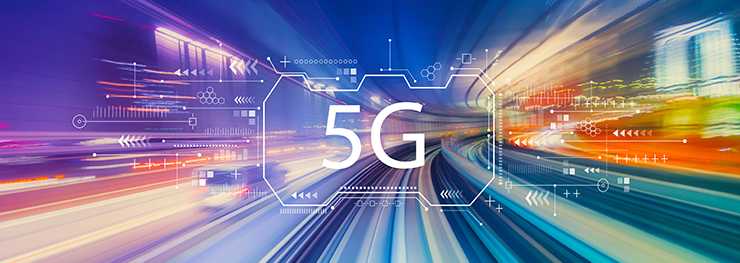
Your Network's Edge®
Blog Post
You are here
5G – hypothetical hype and realistic reality
Unless you live an incredibly sheltered life, you have probably heard a lot about how great 5G is going to be. And unless you are incredibly naïve, you probably don’t believe a word of it.
In this blog post I’ll try to separate the hypothetical from the realistic.
Speed limits
Most of the recent hype has been about pure speed. 5G vows to deliver between 10 and 100 times higher data rates than 4G ever could. If 4G, on a really good day, could deliver 100 Mbps, then 5G will supposedly give you a Gbps. Of course both of these numbers assume that you have climbed the cell tower, glued your phone onto the antenna, and done this at 2 AM when everyone else is sleeping. But it’s the ratio that counts – if today you are getting 50 Mbps from 4G, then you could realistically expect 500 Mbps.
How does 5G attain these speeds, and why would we even want them?
Let’s start with the why. If LTE is enough to support multiple simultaneous video streams, why would anyone want more? The cynical reason is the marketing message. If 5G couldn’t claim a speed up of 10-100 times, then no-one would bother calling it a new generation. 3G promised 10 Mbps as compared to 2G’s 100 kbps, a 100 fold improvement! 4G maxes out at 100 Mbps or so – merely one order of magnitude. Natural evolution of 4G LTE to LTE-Advanced may provide 300 Mbps, but a factor of 3 does not a new generation make.
The less obvious answer is that these higher speeds will enable completely new applications. 1G was the generation which enabled making a phone call without a phone cord. 2G made that phone call more available, affordable, and reliable (and added text messaging as an afterthought). 3G added real data (although the phone screens were too small to fully utilize it) and higher quality audio (although this was rarely turned on). 4G finally enabled the smartphone and true Internet access. The device was no longer a “phone” it morphed into a personal platform for connected applications. 5G makes the final step (at least so far ...) – the devices are no longer phones – smart or otherwise. They may be tightly coupled to people, such as VR/AR glasses, connected pacemakers, or wearable sports trackers, but more often they are not, such as thermostats, refrigerators, coffee pots, drones, electric meters, autonomous cars, traffic lights, and robots. And being the Internet of Things all of these devices are incessantly chatting with each other. Imagine a smart refrigerator continually checking the expiration dates on RFIDed milk cartons, when it discovers a suspect one it automatically compares prices at nearby supermarkets, orders a new carton, tracks the new carton as it is loaded onto a 5G connected drone and flies to the neighborhood, commands the smart window to open, and the smart robot arm to discard the old carton and place the new one on the appropriate shelf. A moment later you reach in and pull out the new carton without ever realizing what just happened.
Technically, 5G’s huge data capacity is not enough to facilitate all of this magic. 5G also needs to support higher connection density than 4G, up to a million connected devices per square kilometer.
Now the how. It isn’t enough to increase the speed of the air interface between the connected device and the base station transceiver (yes, I know radio waves don’t need air, or even aether, but reflect on the expression on the air), we need to increase the capacity of the entire transport network and the mobile core as well. The air interface is souped up by improving the modulation’s spectral efficiency (by about a factor of 3), using more antennas (a factor of 8 or so – certainly more than 2, maybe up to 16), using more but smaller cells (both decreasing the distance from device to base-station, and decreasing the number of devices per base-station), and transmitting at higher radio frequencies where more spectral real-estate is available (a factor of 10 to 20). Taken together, a factor of 10 is undemanding, and even 100 is not overly challenging.
For the backhaul segment, which for 4G utilized Gbps links, the increased capacity means upgrading to 10G or even 100G links. The latest trend is based on a relatively new 25G standard rate, and bonding N of these together. The mobile core will need multiple 100G links, and work is in progress in upgrading the 100GbE standard to 200G and 400G.
I can’t wait
Pure speed measured in Mbps has received most of the attention in publications aimed at the public at large, but it is not the only, and perhaps not even the greatest, challenge. We already mentioned the enhanced connection density, but more important than that is another form of speed – speed measured in the amount of time it takes for a message to travel from the connected device to whichever human, device, or server which needs to receive it. We’ll call this (one-way) latency; and of course we could similarly study the latency in the opposite direction (from the human, device, or server to the connected device), or the round-trip latency. 5G vows to significantly reduce the latency.
How does 5G attain this speed-up, and why would we even want it?
At first thought latency reduction sounds impossible. Don’t radio waves travel at the speed of light – a speed that Einstein famously proved can’t be exceeded? On the other hand, this is obviously not the speed at which digital messages travel. All of us have seen overseas television reporters nodding their heads back and forward before replying to a question which we have already heard. The circumference of the earth is about 40,000 km, so the longest span between anchor and reporter never exceeds 20,000 km which corresponds to 67 milliseconds of radio delay. Even at the slower speed of light in optical fiber, that maximum propagation delay on earth does not exceed a tenth of a second, not the many seconds we experience on the evening news. So it is obvious that propagation latency is not the only, or even the most significant, contribution to latency.
The source of additional (non-propagation) delay is queuing – the amount of time a message waits in queue for its turn to be transmitted. Actually there are many types of queuing delay, of which we will discuss three - acknowledgement hanging, traffic scheduling, and head-of-line blocking.
Acknowledgement hanging occurs when a message waits until receipt of a previous message to the same destination has been acknowledged. It makes little sense to continue sending messages if you are not sure that they are being received (in old one-way radio conversations, one side would say “Over” and wait for the other to say “Roger” - the code for “Received”). If the acknowledgements are delayed, then the transmission hangs until they are received. (This is why TCP transmission rate depends on the latency – a TCP transmitter will only send a certain number of bytes before hanging for acknowledgement.) The 5G air interface has new mechanisms (e.g., the self-contained integrated subframe) whose purpose is to speed up acknowledgement delivery. Furthermore, Mobile Edge Computing, but putting intelligence close to the connected device, is an essential ingredient in speeding up acknowledgements at the application layer.
Traffic scheduling is the methodology a packet forwarding device utilizes to decide which packet to transmit next; for example, a high-priority packet may be selected for transmission before a low priority one, even if the latter has been waiting longer. In fact, low priority packet transmission may even be starved if there are numerous high priority packets to server first. 5G furnishes weapons to combat scheduling delay. It defines end-to-end network slicing, wherein different applications are served by virtual networks that are configured to seem to be completely independent. 5G backhauling will have to employ Time Sensitive Networking technologies, which can guarantee latency upper bounds, even when most of the traffic has latency requirements.
Head of line blocking occurs when the packet selected to be transmitted next has to wait for the packet currently being transmitted to finish exiting the output port. No scheduling magic can eliminate this source of delay, which can be the most significant latency contribution for high priority packets. However, with migration to higher data rates head-of-line blocking times are reduced, and TSN defines “frame preemption” wherein a large packet being transmitted can be pre-empted in favor of a packet requiring expedited forwarding. For high data rates this mechanism is mostly useful for ensuring that non-expedited traffic is not starved.
You can rely on it
Another characteristic of 5G which has not received a lot of press is reliability. While wired telephones are famous for their five nines availability, until now everyone has been extremely tolerant of cellular service drops. Sorry, I entered an elevator and the call dropped. I’m entering a tunnel, I’ll call you back. Sorry, calls always drop when I get here, there must be a building that blocks the signal.
This may have been reasonable when the main device is a smartphone providing mainly entertainment and non-mission critical services, but is unacceptable if Industrial IoT devices miss messages and lead to shutting down of assembly lines. It becomes life threatening when a connected vehicle doesn’t receive a forward collision or emergency brake warning.
The 5G air interface has been enhanced with mechanisms for guaranteed air time, upgraded error correction codes, improved coverage through massive MIMO and device tracking/beamforming, and reduced session drops due to smaller cells and better handoffs between cells. Self-organizing/self-optimizing network technologies will play a major roll, and a new protection switching method known as Frame Replication and Elimination can increase the reliability of the backhaul (at the price of increasing overhead).
What’s next?
I hope that I have convinced you that a lot of what you have heard about 5G is both technologically plausible and has real-life application. The higher data rates, enhanced connection densities, lower latencies, and higher reliability all enable real applications, and are all technologically realizable.
Note that I didn’t say that they are all realizable at the same time, nor that they can all be achieved without paying some price.
In the next installment we’ll dive a bit deeper into the tradeoffs and costs.
About RAD's Blog
We’ll be blogging on a wide range of hot topics affecting service providers and critical infrastructure network operators. Our resident experts will be discussing vCPE, Cyber Security, 5G, Industrial IoT and much, much more.